Muscle dysfunction often occurs in patients with chronic obstructive pulmonary diseases (COPD) and affects ventilatory and non-ventilatory skeletal muscles. We have previously reported that hypercapnia (elevated CO2 levels) causes muscle atrophy through the activation of the AMPKα2-FoxO3a-MuRF1 pathway. In the present study, we investigated the effect of normoxic hypercapnia on skeletal muscle regeneration. We found that mouse C2C12 myoblasts exposed to elevated CO2 levels had decreased fusion index compared to myoblasts exposed to normal CO2. Metabolic analyses of C2C12 myoblasts exposed to high CO2 showed increased oxidative phosphorylation due to increased fatty acid oxidation. We utilized the cardiotoxin-induced muscle injury model in mice exposed to normoxia and 10% CO2 for 21 days and observed that muscle regeneration was delayed. High CO2-delayed differentiation in both mouse C2C12 myoblasts and skeletal muscle after injury and was restored to control levels when cells or mice were treated with a carnitine palmitoyltransfearse-1 (CPT1) inhibitor. Taken together, our data suggest that hypercapnia leads to changes in the metabolic activity of skeletal muscle cells, which results in impaired muscle regeneration and recovery after injury.
www.ncbi.nlm.nih.gov
Loss of skeletal muscle mass is a feature of muscle dysfunction in patients with pulmonary diseases, such as COPD (Debigare et al., 2001; Wust and Degens, 2007; Gea et al., 2016; Barreiro and Jaitovich, 2018). The regulation of muscle mass is thought to result from the balance between protein synthesis and degradation. However, there is evidence that cell turnover is also involved in muscle growth and maintenance of muscle mass. Addition of new myonuclei due to the fusion of satellite cells, the stem cells of skeletal muscle, into pre-existing myotubes play a central role in muscle regeneration (Charge and Rudnicki, 2004; Yin et al., 2013). Hypercapnia is associated with worse outcomes in patients with COPD (Soler-Cataluna et al., 2005; Steer et al., 2010; Yang et al., 2015; Fazekas et al., 2018). We have previously reported that high CO2 induces muscle atrophy, through the activation of the E3 ubiquitin ligase via AMPKα2-FoxO3a-MuRF1 (Jaitovich et al., 2015). The main finding of the present study is that high CO2 also negatively affects muscle regeneration by impairing expansion, differentiation, and fusion of satellite cells into myotubes. We found that impaired differentiation was due to high CO2-induced AMPK phosphorylation and consequent increased fatty acid oxidation as the use of the CPT1 inhibitor etomoxir prevented it both in vitro and in vivo.
Satellite cells are responsible for regulating skeletal muscle repair by proliferating and differentiating into fusion-competent myoblasts and facilitating myofiber regeneration following injury (Lepper et al., 2011; Murphy et al., 2011; Tidball, 2011; Laumonier and Menetrey, 2016). Although our study has the limitation of not using primary satellite cells, we found that high CO2 decreased the proliferation rate of C2C12 myoblasts concording with a previous report in lung epithelial cells and fibroblasts (Vohwinkel et al., 2011). These results suggest a maladaptive effect of high CO2 in cell proliferation via cell-type specific mechanism, particulary targeting mitochondria, as in epithelial cells and fibroblasts, we found a decreased mitochondrial respiration (Vohwinkel et al., 2011), whereas in the present study, we found increased mitochondrial respiration in myoblasts. We also observed that C2C12 myoblasts exposed to high CO2 had decreased fusion index leading to impaired satellite cell differentiation, as highlighted by decreased MHC and NaK-ATPase α2 protein expression (Ladka and Ng, 2000; Tsukamoto et al., 2018). However, not all differentiation markers were affected as myogenin levels were not changed after high CO2 exposure, concordantly with previous publications reporting that not all markers of differentiation get modified in satellite cells with impaired differentiation (Fukawa et al., 2016). In agreement with previous publications, the impairment in proliferation and differentiation of C2C12 myoblasts was independent of cell death or pH (Briva et al., 2007; Vohwinkel et al., 2011; Bharat et al., 2020).
Changes in metabolism have been implicated in impaired satellite cells differentiation during cancer-mediated cachexia (Fukawa et al., 2016; Ceco et al., 2017). CO2 is a by-product of oxidative metabolism, and there are indications that high CO2 level has metabolic effects on epithelial and fibroblast cells (Vohwinkel et al., 2011). We performed metabolic profiling of C2C12 myoblasts exposed to normal CO2, compared them to myoblasts exposed to high CO2 for 3 days, and found that myoblasts exposed to high CO2 had changes in the TCA cycle, in agreement with our previous publication (Vohwinkel et al., 2011). Interestingly, we found an upregulation of carnitine and carnitine-derivatives. Moreover, we found this to be translated into increased mitochondrial respiration with an increase in oxygen consumption linked to ATP production and maximal respiration capacities. Increased fatty acid oxidation by satellite cells has been shown to be involved in the development of sarcopenia (Baraibar et al., 2016) and cancer-induced cachexia (Fukawa et al., 2016), both characterized by impaired satellite cell function. Our data suggest that high CO2 levels cause increased fatty acid oxidation in myoblasts, which could lead to impaired satellite cell function and muscle dysfunction, as seen in COPD patients. For instance, a significantly higher mitochondrial capacity is observed in the diaphragm muscle of patients with COPD as compared with control subjects (Levine et al., 2002).
AMPK is recognized as the cellular energy sensor and metabolic regulator. It has been linked to the pathogenesis of muscle atrophy, through augmented myofibrillar protein degradation by muscle atrophy F-box (MAFbx) and MuRF1 expression (Nakashima and Yakabe, 2007) and FoxO3a transcription factor-induced autophagy (Sanchez et al., 2012). We have reported that high CO2 activates AMPK in lung epithelial cells and myotubes (Vadasz et al., 2008; Jaitovich et al., 2015), and in the present report, we observed a high CO2-induced activation of AMPK in C2C12 myoblasts. ACC plays an essential role as a regulator of fatty acid metabolism by catalyzing acetyl-CoA carboxylation to form malonyl-CoA (Fullerton et al., 2013). ACC is a substrate of AMPK leading to the suppression of ACC activity (Carling et al., 1987), which leads to decreased levels of Malonyl CoA, an intermediate molecule, and an indirect negative modulator of carnitine palmitoyltransferase 1 (CPT1). CPT1 is a mitochondrial outer membrane enzyme that conjugates long chain fatty acyl CoAs to carnitine and facilitates uptake into the mitochondrial matrix for oxidation (Wakil and Abu-Elheiga, 2009). We not only found AMPK phosphorylation in C2C12 myoblasts exposed to high CO2 but also increased ACC phosphorylation, suggesting a mechanism by which high CO2 might increase fatty acid metabolism, leading to satellite cell dysfunction. AMPK seems to be a common denominator in high CO2-mediated muscle dysfunction. With regards to muscle regeneration, AMPKα1 plays a prominent role in regulating satellite cell dynamics, with AMPKα2 playing a potentially more important role in regulating muscle degradation during atrophy (Thomson, 2018). AMPK is activated in response to an increase in intracellular AMP and ADP levels that occurs in response to a fall in ATP. However, AMPK is also activated by an increase in intracellular calcium ions, which is mediated by Ca2+/calmodulin-dependent kinase kinase β (CaMKK-β; Steinberg and Carling, 2019). We have previously shown that high CO2-induced AMPK activation was due to increased intracellular Ca2+ in alveolar epithelial cells (Vadasz et al., 2008), making it a possible mechanism in C2C12 myoblasts, as we found increased mitochondrial respiration.
We demonstrated that high CO2 increased fatty acid oxidation in satellite cell and dysfunction by pre-treating the C2C12 myoblasts with the CPT1 inhibitor etomoxir (Lopaschuk et al., 1988). Etomoxir prevented both high CO2-increased mitochondrial respiration as well as decreased myoblast fusion in agreement with the work of Fukawa et al. (2016) in cancer-induced cachexia. Moreover, we found etomoxir to be an effective inhibitor in vivo when using the cardiotoxin-mediated skeletal muscle injury model. Recently, several reports have challenged the specificity of etomoxir as a CPT1 inhibitor (O’Connor et al., 2018; Raud et al., 2018); therefore, further investigation in the role of fatty acid metabolism in C2C12 exposed to high CO2 levels is warranted.
We found that the mice exposed for up to 21 days to high CO2 not only had decreased CSA (Jaitovich et al., 2015) but also increased the number of centralized nuclei. Centralized nuclei are markers of muscle diseases and delayed repair (Griffin et al., 2010; Forcina et al., 2020). Cardiotoxin causes muscle damage affecting both CSA and centralized nuclei (Ceco et al., 2014), and we found that mice exposed to high CO2 recovered slower after cardiotoxin damage, reflected by decreased myofiber CSA and increased the number of centralized nuclei. Interestingly, intraperitoneal etomoxir was enough to restore the hypercapnia-induced effects on muscle regeneration, given by normalization of centralized nuclei; however, there was an absence of recovery of the CSA, which might be explained by the persistent atrophy induced by proteolysis mediated activation of E3 Ligases of mature myotubes (Jaitovich et al., 2015). These data are suggestive of the pleiotropic effects of high CO2 on muscle pathophysiology: it causes skeletal muscle atrophy through the activation of the E3 ubiquitin ligase MuRF1 via AMPKα2-FoxO3a (Jaitovich et al., 2015) and delays regeneration via the inhibition of proliferation and differentiation of satellite cells.
In summary, we provide evidence that high CO2 impairs skeletal muscle myoblasts differentiation by increased fatty acids oxidation and mitochondrial respiration overload. Accordingly, we propose a mechanism by which a subset population of COPD patients with hypercapnia might have impaired skeletal muscle recovery after injury. A better understanding of these mechanisms might inform novel therapies to prevent and improve skeletal muscle atrophy in COPD patients to decrease morbidity and improve quality of life.
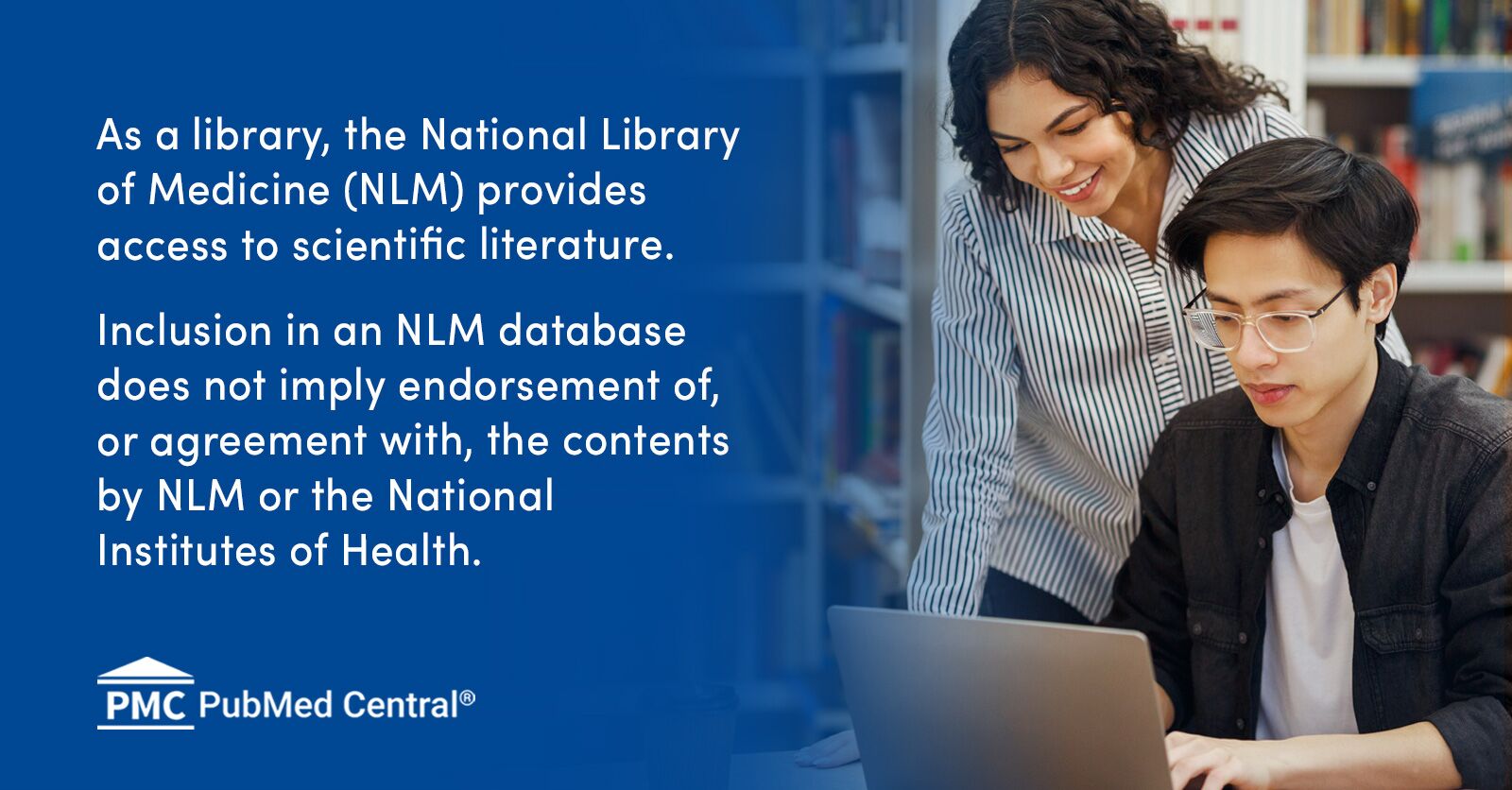
Elevated CO2 Levels Delay Skeletal Muscle Repair by Increasing Fatty Acid Oxidation
Muscle dysfunction often occurs in patients with chronic obstructive pulmonary diseases (COPD) and affects ventilatory and non-ventilatory skeletal muscles. We have previously reported that hypercapnia (elevated CO[2] levels) causes muscle atrophy through ...
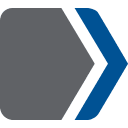
Loss of skeletal muscle mass is a feature of muscle dysfunction in patients with pulmonary diseases, such as COPD (Debigare et al., 2001; Wust and Degens, 2007; Gea et al., 2016; Barreiro and Jaitovich, 2018). The regulation of muscle mass is thought to result from the balance between protein synthesis and degradation. However, there is evidence that cell turnover is also involved in muscle growth and maintenance of muscle mass. Addition of new myonuclei due to the fusion of satellite cells, the stem cells of skeletal muscle, into pre-existing myotubes play a central role in muscle regeneration (Charge and Rudnicki, 2004; Yin et al., 2013). Hypercapnia is associated with worse outcomes in patients with COPD (Soler-Cataluna et al., 2005; Steer et al., 2010; Yang et al., 2015; Fazekas et al., 2018). We have previously reported that high CO2 induces muscle atrophy, through the activation of the E3 ubiquitin ligase via AMPKα2-FoxO3a-MuRF1 (Jaitovich et al., 2015). The main finding of the present study is that high CO2 also negatively affects muscle regeneration by impairing expansion, differentiation, and fusion of satellite cells into myotubes. We found that impaired differentiation was due to high CO2-induced AMPK phosphorylation and consequent increased fatty acid oxidation as the use of the CPT1 inhibitor etomoxir prevented it both in vitro and in vivo.
Satellite cells are responsible for regulating skeletal muscle repair by proliferating and differentiating into fusion-competent myoblasts and facilitating myofiber regeneration following injury (Lepper et al., 2011; Murphy et al., 2011; Tidball, 2011; Laumonier and Menetrey, 2016). Although our study has the limitation of not using primary satellite cells, we found that high CO2 decreased the proliferation rate of C2C12 myoblasts concording with a previous report in lung epithelial cells and fibroblasts (Vohwinkel et al., 2011). These results suggest a maladaptive effect of high CO2 in cell proliferation via cell-type specific mechanism, particulary targeting mitochondria, as in epithelial cells and fibroblasts, we found a decreased mitochondrial respiration (Vohwinkel et al., 2011), whereas in the present study, we found increased mitochondrial respiration in myoblasts. We also observed that C2C12 myoblasts exposed to high CO2 had decreased fusion index leading to impaired satellite cell differentiation, as highlighted by decreased MHC and NaK-ATPase α2 protein expression (Ladka and Ng, 2000; Tsukamoto et al., 2018). However, not all differentiation markers were affected as myogenin levels were not changed after high CO2 exposure, concordantly with previous publications reporting that not all markers of differentiation get modified in satellite cells with impaired differentiation (Fukawa et al., 2016). In agreement with previous publications, the impairment in proliferation and differentiation of C2C12 myoblasts was independent of cell death or pH (Briva et al., 2007; Vohwinkel et al., 2011; Bharat et al., 2020).
Changes in metabolism have been implicated in impaired satellite cells differentiation during cancer-mediated cachexia (Fukawa et al., 2016; Ceco et al., 2017). CO2 is a by-product of oxidative metabolism, and there are indications that high CO2 level has metabolic effects on epithelial and fibroblast cells (Vohwinkel et al., 2011). We performed metabolic profiling of C2C12 myoblasts exposed to normal CO2, compared them to myoblasts exposed to high CO2 for 3 days, and found that myoblasts exposed to high CO2 had changes in the TCA cycle, in agreement with our previous publication (Vohwinkel et al., 2011). Interestingly, we found an upregulation of carnitine and carnitine-derivatives. Moreover, we found this to be translated into increased mitochondrial respiration with an increase in oxygen consumption linked to ATP production and maximal respiration capacities. Increased fatty acid oxidation by satellite cells has been shown to be involved in the development of sarcopenia (Baraibar et al., 2016) and cancer-induced cachexia (Fukawa et al., 2016), both characterized by impaired satellite cell function. Our data suggest that high CO2 levels cause increased fatty acid oxidation in myoblasts, which could lead to impaired satellite cell function and muscle dysfunction, as seen in COPD patients. For instance, a significantly higher mitochondrial capacity is observed in the diaphragm muscle of patients with COPD as compared with control subjects (Levine et al., 2002).
AMPK is recognized as the cellular energy sensor and metabolic regulator. It has been linked to the pathogenesis of muscle atrophy, through augmented myofibrillar protein degradation by muscle atrophy F-box (MAFbx) and MuRF1 expression (Nakashima and Yakabe, 2007) and FoxO3a transcription factor-induced autophagy (Sanchez et al., 2012). We have reported that high CO2 activates AMPK in lung epithelial cells and myotubes (Vadasz et al., 2008; Jaitovich et al., 2015), and in the present report, we observed a high CO2-induced activation of AMPK in C2C12 myoblasts. ACC plays an essential role as a regulator of fatty acid metabolism by catalyzing acetyl-CoA carboxylation to form malonyl-CoA (Fullerton et al., 2013). ACC is a substrate of AMPK leading to the suppression of ACC activity (Carling et al., 1987), which leads to decreased levels of Malonyl CoA, an intermediate molecule, and an indirect negative modulator of carnitine palmitoyltransferase 1 (CPT1). CPT1 is a mitochondrial outer membrane enzyme that conjugates long chain fatty acyl CoAs to carnitine and facilitates uptake into the mitochondrial matrix for oxidation (Wakil and Abu-Elheiga, 2009). We not only found AMPK phosphorylation in C2C12 myoblasts exposed to high CO2 but also increased ACC phosphorylation, suggesting a mechanism by which high CO2 might increase fatty acid metabolism, leading to satellite cell dysfunction. AMPK seems to be a common denominator in high CO2-mediated muscle dysfunction. With regards to muscle regeneration, AMPKα1 plays a prominent role in regulating satellite cell dynamics, with AMPKα2 playing a potentially more important role in regulating muscle degradation during atrophy (Thomson, 2018). AMPK is activated in response to an increase in intracellular AMP and ADP levels that occurs in response to a fall in ATP. However, AMPK is also activated by an increase in intracellular calcium ions, which is mediated by Ca2+/calmodulin-dependent kinase kinase β (CaMKK-β; Steinberg and Carling, 2019). We have previously shown that high CO2-induced AMPK activation was due to increased intracellular Ca2+ in alveolar epithelial cells (Vadasz et al., 2008), making it a possible mechanism in C2C12 myoblasts, as we found increased mitochondrial respiration.
We demonstrated that high CO2 increased fatty acid oxidation in satellite cell and dysfunction by pre-treating the C2C12 myoblasts with the CPT1 inhibitor etomoxir (Lopaschuk et al., 1988). Etomoxir prevented both high CO2-increased mitochondrial respiration as well as decreased myoblast fusion in agreement with the work of Fukawa et al. (2016) in cancer-induced cachexia. Moreover, we found etomoxir to be an effective inhibitor in vivo when using the cardiotoxin-mediated skeletal muscle injury model. Recently, several reports have challenged the specificity of etomoxir as a CPT1 inhibitor (O’Connor et al., 2018; Raud et al., 2018); therefore, further investigation in the role of fatty acid metabolism in C2C12 exposed to high CO2 levels is warranted.
We found that the mice exposed for up to 21 days to high CO2 not only had decreased CSA (Jaitovich et al., 2015) but also increased the number of centralized nuclei. Centralized nuclei are markers of muscle diseases and delayed repair (Griffin et al., 2010; Forcina et al., 2020). Cardiotoxin causes muscle damage affecting both CSA and centralized nuclei (Ceco et al., 2014), and we found that mice exposed to high CO2 recovered slower after cardiotoxin damage, reflected by decreased myofiber CSA and increased the number of centralized nuclei. Interestingly, intraperitoneal etomoxir was enough to restore the hypercapnia-induced effects on muscle regeneration, given by normalization of centralized nuclei; however, there was an absence of recovery of the CSA, which might be explained by the persistent atrophy induced by proteolysis mediated activation of E3 Ligases of mature myotubes (Jaitovich et al., 2015). These data are suggestive of the pleiotropic effects of high CO2 on muscle pathophysiology: it causes skeletal muscle atrophy through the activation of the E3 ubiquitin ligase MuRF1 via AMPKα2-FoxO3a (Jaitovich et al., 2015) and delays regeneration via the inhibition of proliferation and differentiation of satellite cells.
In summary, we provide evidence that high CO2 impairs skeletal muscle myoblasts differentiation by increased fatty acids oxidation and mitochondrial respiration overload. Accordingly, we propose a mechanism by which a subset population of COPD patients with hypercapnia might have impaired skeletal muscle recovery after injury. A better understanding of these mechanisms might inform novel therapies to prevent and improve skeletal muscle atrophy in COPD patients to decrease morbidity and improve quality of life.